Innovation out of the Wind Tunnel: Drive Drag Reductions in Motorsports to the Top with Riblet Surface Applications
Task
Increasing the efficiency of racing vehicles with Riblet surfaces.
Solution
Performing wind tunnel tests with different Riblet arrangements based on detailed computational fluid dynamic Riblet analyses.
Benefit
Efficiency increases directly convert to a reduced fuel consumption, higher average and top speeds and therefore significantly faster lap times.
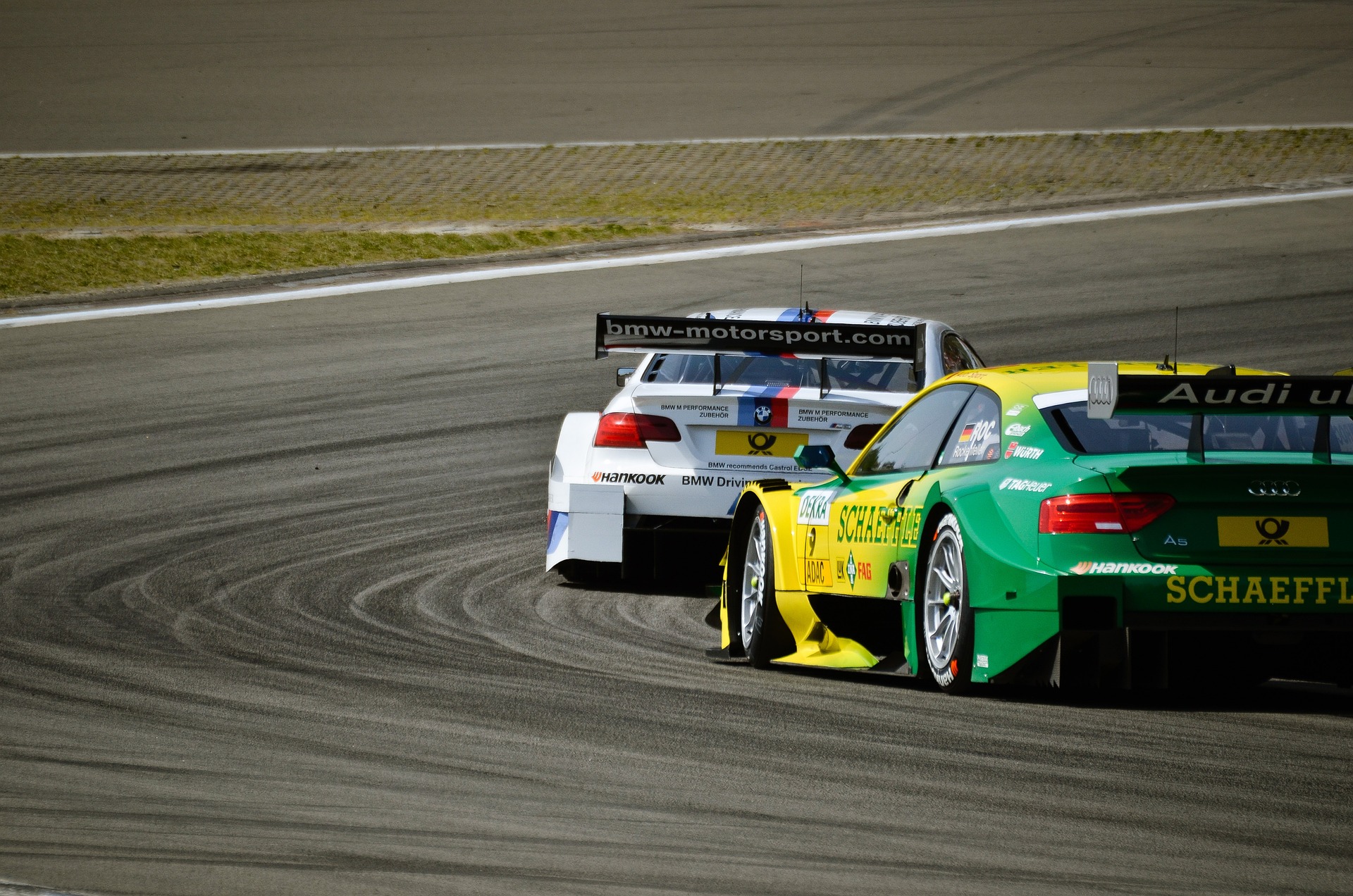
Figure 1: High Performance Racing vehicles of the “DTM - Deutsche Tourenwagen Masters”
Wind tunnel testing in motorsports
Motorsport is a fascinating world that is constantly evolving and offering new technological, aerodynamic and strategic challenges. From the early days of automobile racing to modern high-performance racing cars and motorcycles, motorsport has continuously evolved and remains a dynamic environment for innovation and excellence. Crucial elements for the technological progress in motorsports, which became indispensable in the recent past and still face growing importance, are wind tunnel experiments. Wind tunnels provide valuable insights into the aerodynamics of vehicles, which enables engineers to potentially enhance performance and increase competitiveness. The basic and general configuration of a wind tunnel consists of a long tube with inlet and outlet. The testing area with the model is positioned in the tunnel’s center. Fan equipment generates a controlled air flow, which creates the required testing conditions. Specific measuring instruments record the flow parameters, as well as other relevant data. This enables further analyses and optimization of the model’s aerodynamic behavior.
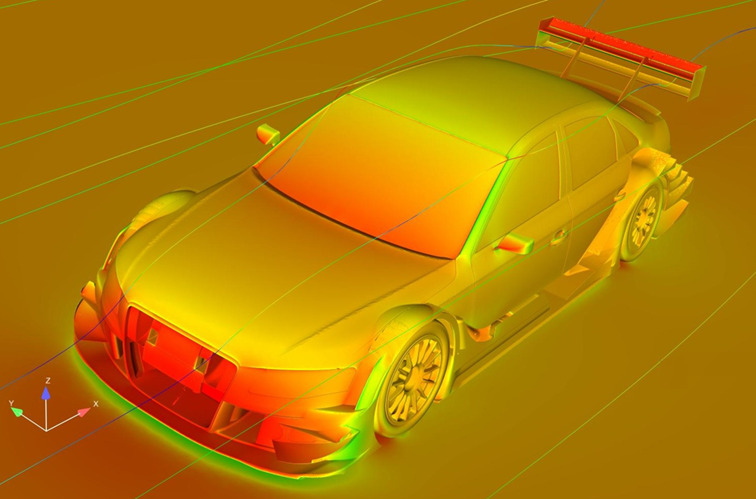
Figure 2: Visualization of Flow Parameters of a Race Car through advanced CFD Simulations
A wind tunnel is a controlled environment that allows to simulate real-world conditions and study the interaction between air and a racing vehicle's surfaces. This process is pivotal in optimizing the design and performance of race cars and motorcycles, ensuring they can attain maximum speed, stability, and handling on the track. The underlying concept of a wind tunnel is the Reynold resemblance, which states, that flow conditions occurring around two similar geometries with the same Reynolds number, are similar. Therefore, if the Reynolds number of a real vehicle or aircraft and of a downscaled model are identical, the flow around both will have similar characteristics. Although the Reynolds number depends on the flow velocity, the fluid’s viscosity, and on geometric parameters, also other conditions can influence the validity of such scaled experiments.
Essential not only for modern engineering and aerodynamics, but also for the design and conduction of valid wind tunnel tests, are numerical analyses. Computational Fluid Dynamic (CFD) simulations are powerful tools to configure realistic testing conditions and to overcome cost and time consuming trial and error loops. Furthermore, CFD simulations are valuable tools to validate wind tunnel tests and vice versa. With our in-house wind tunnel, which is primarily designed to test Riblet applications on small scale air foils, we put a strong focus on combining experimental and numerical analyses to obtain information of high significance in this sense. Figure 3 for instance shows the flow visualization of an airfoil’s suction side. At the leading edge, the flow is laminar, while a transition zone develops in the wing’s center. Towards the trailing edge, the flow shows turbulence. This finding is also confirmed by the CFD simulation, which shows the low wall shear stress in the area of the transition zone. Conformity of the experiment and the simulation are given, as a comparison of the images shows.

Figure 3: Flow visualization of a motorsport airfoil in the wind tunnel and a CFD simulation of the wall shear stresses of the respective airfoil
Riblets in the wind tunnel
Wind tunnel tests are used to analyze the effects of Riblet surface applications and to demonstrate their benefits, for instance on race car air wings. The experiments at our in-house wind tunnel mainly investigate different Riblet arrangements regarding their influence on drag and flow turbulence. Precise measurements and simulations are also used to determine which Riblet configuration offers the highest performance gains. This enables the development of innovative aerodynamic solutions. Riblet structures interact with the turbulent boundary layer and hereby increase lift and reduce fluid-dynamic drag. Decreasing the cross flow also leads to an enhanced flow stability and delayed stall. The aerodynamic behavior improves and the performance benefits are significant. Figure 4 shows the increased downforce with applied Riblet surfaces, measured in our wind tunnel. The tests of the airfoil were conducted with and without Riblets for different angles of attacks.

Figure 4: Increase of Downforce and Decrease of Drag on a Racecar’s Air Wing with shark skin textured surface
Outcome
The advantageous aerodynamic mechanisms of Riblets bring substantial performance increases for the respective use case. Applications on race cars or motorcycles offer efficiency increases, reduced fuel consumption, higher average speeds and top speeds and increased downforce. These effects directly convert to faster lap times. Proved are these effects not only by our numerical analyses and wind tunnel experiments, but also on race track. Major performance gains were for instance demonstrated at the “Deutsche Tourenwagen Masters – DTM” where our Riblet surface applications yielded to up to 0.3 seconds faster lap times. Due to the high performance gains, the application of Riblets was banned in the racing series after a successful long term deployment by different teams.